Аннотация
В работе представлен проект гиперспектрального малого спутника весом около 120 кг. Спутник
предназначен для наблюдения за состоянием трубопровода, раннего обнаружения повреждений и
выработки рекомендаций к их устранению. Планируется, что спутник обеспечит пятиметровое
разрешение на 700 спектральных линиях в диапазоне 0.3 - 1.6 микрон.
Данная работа состоит из двух частей. В первой части описывается малый спутник, который
является улучшенной версией микро-спутника, представленного в 1996г. на Симпозиуме IAA в
Берлине. Для тестирования аппаратуры и проверки идей, которыми руководствовались создатели
при разработке модулей гиперспектрального спутника, были изготовлены бортовые приборы
авиационного базирования. Приборы использовались для мониторинга газовых трубопроводов,
расположенных в северной части России. Летные эксперименты были успешно проведены в августе
и сентябре 2000 и 2001 гг. Во второй части статьи представлены результаты тестирования
бортовой аппаратуры гиперспектрометра. Приведены результаты обработки и анализа полученных
измерений, на основании которого удалось подтвердить правильность выбора характеристик малого
спутника.
Проект разрабатывается при поддержке программы Газпрома Аэрокосмического мониторинга и
Федеральной космической программы Российского аэрокосмического агентства.
Abstract
A project of the hyperspectral small satellite about 120 kg mass based on the modern technology
is presented. The project is dedicated for “Gazprom” pipelines sensing to evaluate their
condition, early recognize failures and make recommendations for preventive service. The
satellite is planned to provide of 5 meter resolution in 700 spectral lines of 0.3 - 1.6
mm
range.
The paper consists of two parts. The first part describes a small satellite which is an
enhanced version of the microsatellite presented at Berlin’s IAA Symposium in 1996. To test
instruments and to check the ideas employed for the development of the satellite’s onboard
hyperspectral unit, an airborne version of the device was fabricated and used for northern
located gas pipelines monitoring. The flight testing is successfully performed on
August-September, 2000 and 2001. In the second part the results of testing of the airborne
version of the hyperspectral instrument are presented. Measurement data have been processed,
analyzed and used to prove the choice of small satellite characteristics.
The small satellite project is developed under support of Gazprom’s Program of Aerospace
Monitoring and the Federal Space Program of the Russian Aerospace Agency.
INTRODUCTION
The Board of JSC “Gazprom” adopted in 2000 the Program of Aerospace
Monitoring. An ultimate goal of the Program is to
make an overall system for the resource monitoring of areas and more than 200
thousand kilometers length of pipelines and also to make a pipeline-inner
diagnostics, ground-level inspection, aero-monitoring
and monitoring with a space segment at the final stage of the development. The
space segment is supposed to be supplied with either conventional system for
the multispectral space sensing in optical range or more sensitive research
equipment for hyperspectral monitoring of production areas, pipelines and of the Earth’s limb. The latter approach
purposes to predict the tectonic processes in the Earth’s crust that is
necessary for estimation of the pipelines geotechnical state.
In 2000 the Russian Aerospace Agency opened a tender for the development
of a SC for the hyperspectral sensing of production
areas (the Winner of this tender is CSDB “Progress”
located in Samara) and another tender for the development of optical
hyperspectral SC for the Earth’s limb exploration (the Winner of this tender is
NIIEM located in Istra City near Moscow). Besides, JSC “Gazprom” develops
together with aerospace industry the project for hyperspectral monitoring of
pipelines which combines the hyperspectral resolution of 1 nm in 700 spectral lines and of 3-5 m spatial resolution, and
precise system of pre-programed scanning of pipelines with narrow angle of
view. The hyperspectral instruments for airborne and space locations are
designed by “Reagent” Research & Development Center (R&DC) in Moscow.
As a first step on the way to
develop the space segment a specialized airborne laboratory has been built
using technology developed by “Reagent” R&DC
by the order of JSC “Gazprom”. This hyperspectrometer sample provides the
spectral resolution within 1.0 - 2.0 nm. The main purpose of the development of
the airborne sample is to estimate the hyperspectral information efficiency
using the objects of JSC “Gazprom”. Achievements in the sensing technology and
testing instruments developed are confirmed by certified test flights over the
gas pipelines near Gryazevets-Myishkin area situated at the European part of
Russia. The tests were successfully carried out in August, 2000 and 2001. The
industrial monitoring of the northern pipelines with employing of the
hyperspectral instruments was successfully carried out in September, 2000 and
2001 by the Corporation “EcoTech-North” by the order of “Yamburggasdobyicha”
and of the Department of Science, New Technology and Ecology of “Gazprom”.
The flying lab has been also supplied apart from the hyperspectral
instruments with conventional instruments for multispectral air photography,
infrared scanner, time-reference system and with the coordinate binding based
on GPS/GLONAS navigation systems. Simultaneously with on-ground exploration of
the pipelines by conventional means, the new approach which uses a geo-radar
for the exploration of the Earth below the ground level was tested.
1. PROBLEMS OF PIPELINE MONITORING
Production, transportation, and processing of gas
render noticeable affect the environment. Qualitative and quantitative
estimations of characteristics of the effect of the environment on objects of
the gas industry and, also, the effect of objects of this industry on the
environment cause the modern progress of the gas complex. In this way, to solve
the following tasks is supposed to be important:
·
The environment of the
gas complex objects can result in emergencies and, therefore, it requires to
execute a scheduled work to recover the complex segments being in
before-emergence condition. This poses a task to monitor the gas complex
objects with the purpose to inspect and forecast the approaching to the
emergency condition.
·
Nowadays the cost of gas
and other mineral products is augmented by the value of penalties and
allocations to recover the ecology of the gas production areas. To avoid
cutting the profitability of the gas complex, the monitoring system has to
estimate the real ecological hazard to minimize the penalties for the
environment recovery. Also, this system should separate the hazard purely made
by the gas complex from that of the other industrial objects and pollutions of
the natural origin. It is necessary to analyze the tendency in the gas complex
which affects the environment with the purpose to prevent violations of the
ecology at earliest stage with minimum efforts.
·
Development of the gas
complex also requires a permanent completion of the operational deposits and to
search for the new ones.
·
To lay pipelines it is
necessary to be familiar with the geological structure of prospective
directions of the pipelines and to evaluate the geoindustrial effects of
pipeline beforehand. It is possible to evaluate such effects analyzing the
progress of the complex already operates.
The tasks listed above correlate with the tasks
arising in other areas of industry. The methods and technological means
developed for that can be successfully used in the close areas of human
activity. It may increase the total value of monitoring.
2. COMPLEX MONITORING OF PIPELINES
AND NOTHERN RESOURCE FIELDS
An example of the combined approach to
arrange the complex monitoring is on-ground and airborne explorations which
were carried out in August and September, 2000 in framework of the Program of
the aerospace and on-ground explorations of the Yamburg Oil-Gas-Condensate
Fields (YOGCF). The interest to the northern territories is stimulated by close
relationship between northern gas papelines and the permafrost soils. This
determines the geotechnical pipeline systems class. The reliability of
pipelines is governed by the mechanism of interaction in these geotechnical
systems.
The set of diagnostic methods is
defined by the features in this problem and, therefore, includes:
·
aerospace
monitoring of the pipelines and territory of fields;
·
landscape,
engineering-geologic exploration;
·
measuring of
parameters of tight-strained state and outside defectoscopy of pipelines;
·
development
of mathematical forecasting models for linking the dynamics of changes in
natural, first of all, permafrost conditions with reliability and the residual
lifetime of pipelines;
·
development
of intelligent systems combining geographical information systems,
technological databases for the analysis, and recommendation blocks.
The complex examinations conducted on
YOGCF territory, are labour-consuming and extra expensive. However, they are
the basis for the development of low-cost and operative approaches engaging the
methods for space monitoring of the Earth.
Since there is a sufficient experience in
combined ground-level and airborne examinations of the local regions, in future
it is possible to ensure operative and low-cost monitoring of all pipeline
network. Also, it is possible to perform reconnaissance and contouring of the
resource fields through a space monitoring of Earth only. In this way the
airborne monitoring is implemented first as a low-cost one and fast to be
implemented, and as an indispensable device in the subsequent embodying of
space monitoring program.
The practice of examination of YOGCF
demonstrates that the monitoring based on the conventional approaches of
monitoring has a limited applicability. The images of the high spatial
resolution, with reference to the linear objects masked by two-meter stratum of
a soil do not give us an essential increase of self-descriptiveness.
Hyperspectral survey containing up to thousand of
spectral channels provides a qualitative raise of the information capacity of
extremely low-cost, in terms of the hardware solution, monitoring in visible
and SWIR bands. The airborne monitoring is a part of a comprehensive diagnostic
Program of northern pipelines of the YOGCF geotechnical system. Diagnostic
program includes the following items:
-
permafrost, landscape,
engineering-geologic examinations;
-
measuring of parameters
of tight-strained state and outer defectoscopy of pipe lines;
-
making of mathematical
prediction models which combine dynamics of variation in natural, first of all,
permafrost conditions with the reliability and life resource of pipelines;
-
making
intelligent systems integrant geographical information systems, technological
databases for the analysis, and recommendation blocks of repair, modes and gas
transportation technologies.
For the examination of gas pipelines and
depositing territories in Yamburg region the following equipment was used:
-
airborne
camera (ABC) such as ТК-10 with 100-mm focus, capture 1,8 Н of flight
and command units for the sight ABC operation control the electronic;
-
solar
reflective hyperspectral device (engineered by “Reagent” R&DC.)
Hyperspectral
instrumentation was designed to monitor spectral characteristics of the
communications and trunks in absorption line strips, direct and diffuse
reflection in near ultraviolet, visual and near infrared spectrum range, in two
polarizations. The spectral decomposition is carried out through diffraction
gratings. The spectral resolution is 1.0-2.0 nm. Field of view of the device is
equal to 12 degrees. The spatial resolution at 1000 m height is about one meter
in a perpendicular to current plane traffic direction and about two meter in
parallel at the plane cruising speed. Hyperspectral instrumentation embedies a
modular approach. Each module is designed for operation in a particular
spectral range and, in average, is provided with 200 spectral channels.
In
year 2000 and 2001 expedition pioneering four such modules enveloping a visible
band of 0.4-0.85 mm were tested. Other four modules covering the band of
0.3-1.8 mm were scheduled in year 2004 expeditions and the gamut
from 0.3 up to 1.6 mm is overlapped. The information received by modules is
converted to the video signal and transmitted to the recording unit. The
received data is fixed to the terrain by the synchronous video data which are
received from service video camera involved in the complex, ABC photo data, SC
navigator, and onboard inertial navigation system.
First,
the complex was proved over tundra, in the land with permafrost, with no woods
and big bushes. Hyperspectral data are clocked by a
common signals and are convenient for the subsequent co-processing.
- Scanning radiometer “AGROS”. The device supplies spectral zoned images
in visual, near and far (8-12 mm) infrared (IR) spectrum bands. The
information of a radiometer ensures recovering “natural” underlying surface
temperature in a range from -40 up to +50 degrees C to within 0.3 degrees.
Resolving ability at the altitude of one kilometer is not worse than one meter.
The strip of capture constitutes 1.4 altitude of flight.
- ABC navigation complex (ABCN). Within a combination of a complex with
GPS and onboard PC-type computer, ABCN allows us to obtain navigational data
and to realize the steering calculations of manual air navigation displaying
them at the screen as it is required (intermediate waypoints, planar
coordinates, data on the rate, velocity and deviation from required course).
The conjugation with ABC allows us to file and to issue by other ABC systems
the moment of actuation ABC locks and also to store them in a computer memory
with binding to coordinates. For the express
analysis the data of video sensing, ABC and AGROS, simple correlation handling
for separate hyperspectral sub-bands are used. The instrumental data of
ground-level surveys of the year 2000 is subject for the lab processing at a
present time.
The
presented sampling of the hyperspectral monitoring data solves a main problem
of pre-processing (i.e. normalization, correlation analysis and centering). The
diagnostic of reference anomalies on trunks are detected. The continuous
processing of the data set is made at present time by standard technique.
The airborne laboratory surveys gas lines made for a series of objects
passing YOGCF territory, trunks of Yamburg - Nida, motorway Yamburg – New
Urengoi, collector and the other infrastructure. The duration of sensing is
more than 30 hours in flight.
3. HYPERSPECTRAL MONITORING DATA PROCESSING
The hyperspectral monitoring data
are recorded on the magnetic tape accompanied by time markers. They permit to
realize their joint binding for further laboratory processing. The received
records are digitized and computer processed. The processing consists of
several stages. At the first stage the hardware effects (i.e. distortion and
inhomogeneity of the sensitivity) are eliminated. The second stage implies the
uniform coordinate binding of the data. At the third stage the hyperspectral
data of correlation processing is performed using allocation maps of
correlation functions which concern the given test field.
For obviousness the joint maps of
correlation with several test fields are made. Thus, the correlation of one
test field is valued till the given functional to a level and at excess of this
level is designed by a pseudocolour which is particular for each test field.
The treatment includes coprocessing
hyperspectral data with other aircraft complex channels, data of ground-level
measurings on the pipe line – permafroze geotechnical system patterns basis.
The illustrative materials demonstrating primary hyperspectral information in
one of modules in a subband 0.45-0.65 mm
are presented in Figures 1-5 in the following consequence [6,7].
Figs.1.1-1.2
show halftone image received by convolution hyperspectral information in a
spectral band 0.45-0.65 mm.
Figs.2.1-2.2 show correlation convolution
(colour image), made on 9 test fields including reference points such that
water, messes, sand, point on the naked pipe line etc. At build-up of figures
the hyperspectral data in each point were set norms per unit, i.e. the
dependence of an integrated reflectivity on a place and requirements of
shooting was eliminated.
Figs. 3.1-3.4 show the same as Fig.2 but
each in one of colors.
Fig.4
demonstrates the image of the sub-aircraft point which is noted by cross-hairs
in the first two figures. The line on the CCD image exhibits field (area) of
synchronous hyperspectral shooting.
Fig.5 shows gray scale image hyperspectral
shooting gauged and normalized on a curve of a gear transmission of the
atmosphere. The vertical axis is spatial coordinate. The horizontal axis is
spectral coordinate in a gamut 0.45-0.65 microns.
4. BASIC TECHNIQUES FOR BUILDING UP
OF THE HYPERSPECTRAL MICRO SATELLITE
The Earth
optical monitoring future is bound to the development of the hyperspectral
observation techniques. The typical spectral resolution of Dl~10 nm of the
operating multispectral optical systems employed now will be replaced with
spectral resolution of Dl~1nm and further down to Dl~0.1nm. Reaching
for a such high spectral resolution gives a steep surge to the equipment
sensitivity requirements or the equivalent expanding of the optical systems
aperture.
Whereas construction of the large aperture
optical instruments with complex systems of image stabilization at the
photo-receiver’s focal plane highly boosts the cost of such systems, increases
the development and start times. Therefore, the major trend in the Earth monitoring
equipment designing in terms of commercial use is finding the way to reduce the
size, mass and power consumption parameters as well as to considerably lessen
the requirements for the SC platform stabilization. The on-line flexibility and
globalness of the observations are not the obligatory factors in the commercial
use. This allows us to operate the optical electronic system according to the
user request (e.g. via Internet) pointing out the local observation area and
the specific spectral lines (or their suspension) at which the observation
should be made. Therewith one may evade the high redundancy degree of the
conventional observations.
The
practical realization of this ideology can be based on the two technological
achievements. They are programmable acousto-optical filter and separate photons
counting device with the high space-time resolution [1]. Principles of the hyperspectral
observation technique are illustrated on Fig.6.
4.1. Acousto-Optical Tunable Filters for Visible and
UV Lights
Acousto-optical tunable filters (AOTF) with
optical size of approximately
8-10 mm for visible and ultraviolet spectrum on the base of TeO2 and
KH2PO4
(denoted by KDP) crystals are developed and manufactured by “Reagent” R&DC.
These devices have the highest space, frequency and time simultaneous
resolutions. The spectral resolution is better than one nm. The frequency band width of both filters is 55-90 MHz that
allows us to use only one light frequency generator with a row of different
power amplifiers. Acoustic power level for the AOTF based on TeO2 and
KDP is about 1 W and 5 W respectively. The optical tuning is carried out by
means of software. The first AOTF has been similarly constructed to the one
reported by I.C.Chang. 163°
YZ-cut LiNbO3 transducer was bonded to
the TeO2 crystal with In metal.
The
most significant advantage of this type of AOTF is its low input electric power
required. The optical tuning is carried out by means of software and the filter
randomly permits any wavelength within its spectral range in less then 15 msec.
The KDP crystal has been used in the designing and fabrication of AO cells for
UV spectrum [2,3]. Specifications of the noncollinear AOTF on the KDP crystal
available are as follows:
Spectral range, nm
|
250...400
|
Optical aperture size, mm
|
3...8
|
Angular aperture size, degree
|
0.5...6
|
Input optical polarization
|
Linear
|
Access time, ms
|
2...5
|
RF frequency range, MHz
|
55…130
|
Specifications of the noncollinear AOTF on the
TeO2 available are as follows:
Spectral resolution, nm
|
1...10
|
Spectral range, nm
|
400…2000
|
Spectral resolution, nm
|
1..10
|
Optical aperture size, mm
|
3...8
|
Input optical polarization
|
Linear
|
Access time, sec
|
5..13
|
RF frequency range, MHz
|
50…130
|
The
spatial resolution of AOTF is limited by diffraction and depends on the optical
aperture size.
4.2. The Micro-Channel Plate (MCP) Photon Detectors
The most sensitive detectors known at the
moment are those based on the MCP technology which provide (unlike the
CCD-storing systems) detection of the photon beam in the single photon
sequential analysis mode determining the coordinates at the 2D focal plane with
high spatial resolution along with a high time resolution accessible to
super-speed CCD-systems. The technology of these detectors is based on the
principle of conversion of a weak photon beam into the photo-electron current
from the photo-cathode surface and its subsequent amplification by ~106
factor with the MCP while preserving the spatial-time resolution (with the
accuracy of ~20 mm in
space and 10-9 s in time). Further on one
of the multi anode collector system read-out options of the remitted electron
flow yields the X, Y, t coordinates by the computation unit. The best aerospace
specimen of such systems (MAMA-detector) reaches the 1000 x 1000 pixel of
spatial resolution and the photo-electron counting rate of 106 s-1
under the photo-cathode quantum output of ~10-20%.
One
of this detector version was developed by “Reagent” R&DC [4,5]. It differs from the MAMA-detector by simplified
collector system and more thorough signal processing mathematics. While
properties of the “Reagent” R&DC variant are preserved close to that of the
MAMA, the former can be implemented within one kg mass – one liter volume and
ten watt power consumption. We should note that the detector’s engine noise is
determined by the photo-cathode noise and no cooling is required. In the
framework of this project the rate of photon counting will be increased in
100-1000 times.
4.3. Star-Tracker
The MCP detector is a proper star-tracker due
to high sensitivity and high space-time resolution. Essential factor is that
mathematical conversion of the MCP-detector data may compensate the
star-tracker’s angular motion with sufficient high rate by transferring to the
proper reference system [4]. Highly accurate determination of angular motion of
the optical axis is the result of this procedure. The
high sensitivity of the MCP-detectors allows us to construct a narrow-angle
star-tracker which recognizes the starry sky image and does not require the
basis-system of bright stars.
5. GENERAL CONCEPT OF SCIENTIFIC INSTRUMENTS
ENERGY ESTIMATES
Cutting down the scope angle q0 to
10-3 rad under the extended
effective aperture diameter of the input lens eyes up to 15 cm makes it
possible, as on Fig.6, to sharpen the angular resolution to Dq~10-5 rad
that corresponds at H~500
km to 3-5 m of the spatial resolution, sufficient to solve the ecological
monitoring tasks in terms of the local Earth parts. In this case W0~10-2
W/km×cm2×str
in the visual band, the input telescope effective area S
~225
cm2 , the scope solid angle q0~10-6 rad2, Pqw~10-1,
cAOTF ~1/3,
and assume
Dl~1
nm for effective hyperspectral analysis than the MCP photon counting rate amounts
to:
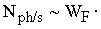   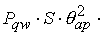  ,
i.e. the photon number is rather small and the photon
counting mode realization is required. An image of 100 brighten grades is
available in the 10 narrow spectral band (or 10 correlation functions which are
equivalent to the final result of the hyperspectral cube processing) from the
1500 ´
1500 m area during time interval t =
100 s.
A detector combined with the star-tracker
provides photon coordinates fixing an accuracy of 5 m with respect to the
ground based reference coordinate. Whereas the detector plane image hold system
can be a rough mechanical circuit with an accuracy of ~10-3 rad
that implies no problem. While the given local plot can be traced by the SC
during ~100
s of flight within the strip width of ~1000 km. This time allows us
to gain the hyperspectral stereo image due to synthesizing of the base during
the flight. The complete system on the base of the today technologies can be
designed within ~4
liter of volume and ~4 kg
of mass with the power consumption of ~15 W. For small
satellite with weight m = 200 kg the number of simultaneously
measured spectral bands can be increased up to 1000 and observed area can be
increased considerably. The number of the image brightness can be increased up
to 500 by means of adaptive approach to using hyperspectrometer resources.
AKNOWLEDGEMENTS
We would like to thank:
Makredenko, A.Victorov, A.Dudkin (Rosaviacosmos, Depatment of Remote Sensing)
for analysis and expertise of the project; C.Kulik, D.Gorovec (Rosaviacosmos)
for collaboration with European Union; A.Ananenkov, E.Dedikov (Gazprom,
Depatment of Science and Technology), Yamburggazdobicha Company for the flight experiments assistance. Also this work is partly
supported by ISTC (project: 23-23) and RBSF (project: 03-01-00-652).
REFERENCES
1.
Rodionov I., Ovchinnikov M., Microsatellite for Remote
Sounding of Environment, Digest of the IAA International Symposium “Small
Satellites for Earth Observation”, 4-8 November, 1996, Berlin, Germany,
pp.225-229.
2.
Rodionov
I., Ponomarev A. at.all,
Active Optical Ecological Monitoring // SPIE Vol. 2107, p.476-494.
3.
Rodionov I., Ponomarev A. at.all, Wide Aperture Acousto-Optic
Turnable Filters for Visible and UV Lights // SPIE Vol.2449, p.200-207.
4.
Rodionov I., Rodionova I., Ovchinnikov M., The Microsatellite for Remote
Exploration of Environment // SPIE,1997, Vol.3119, №3119-11.
5.
Rodionov
I., Rodionova I., Ovchinnikov M., Approaches to Develop the Earth Observing Systems Based on
the Turnable Acousto-Optical Filter and Separate Photons Counter with MCP //
SPIE, 1997, Vol.3117, №3117-10.
6.
Vorontsov
D.V., Orlov A.G. Kalinin A.P., Rodionov A.I., Shilov I.B., Rodionov I.D.,
Lyubimov V.N., Osipov A.F. Usage of the Hyperspectral Measurements
for Remote Sensing of the Earth. Preprint of the Institute for Problems in
Mechanics of RAS, 2002, №702 (in Russian)
7.
Vorontsov
D.V., Orlov A.G. Kalinin A.P., Rodionov A.I., Shilov I.B., Rodionov I.D.,
Lyubimov V.N., Osipov A.F. Sub-pixel Processing of the Hyperspectral
Measurements. Preprint of the Institute for Problems in Mechanics of RAS, 2002,
№703 (in Russian)
8.
Khrenov
N.N., Dmitrievsky A.N., Ananenkov A.G., Sheremet V.V., Ponomarev A.A., Rodionov
I.D. Hyperspectral Aerospace Monitoring of Pipe-lines and Deposit
Areas // Science and Engineering in Gas-Industry, Moscow, 2001, №2-3, pp.37-46
(in Russian)
|